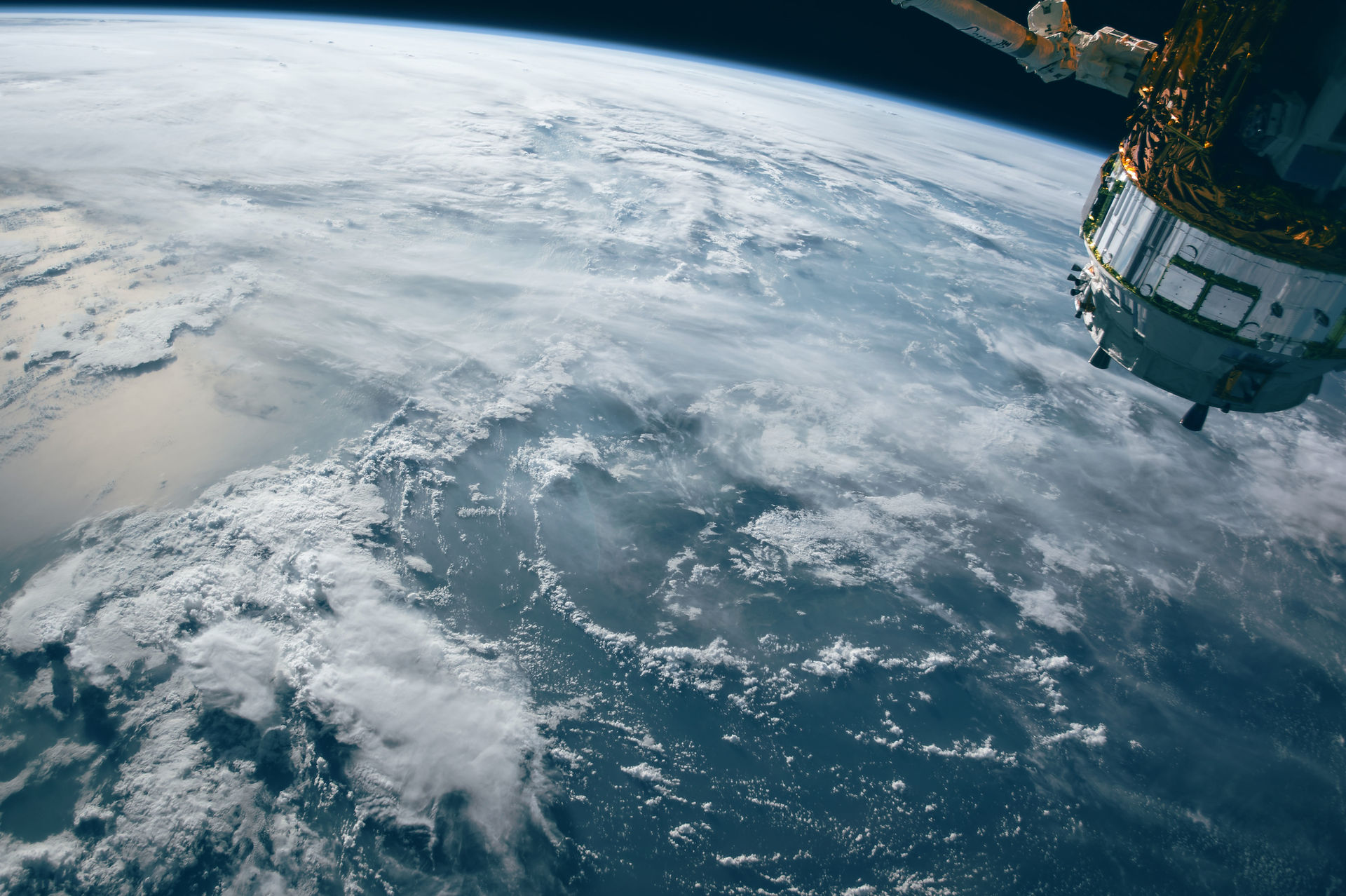
PARTICLE PHYSICS
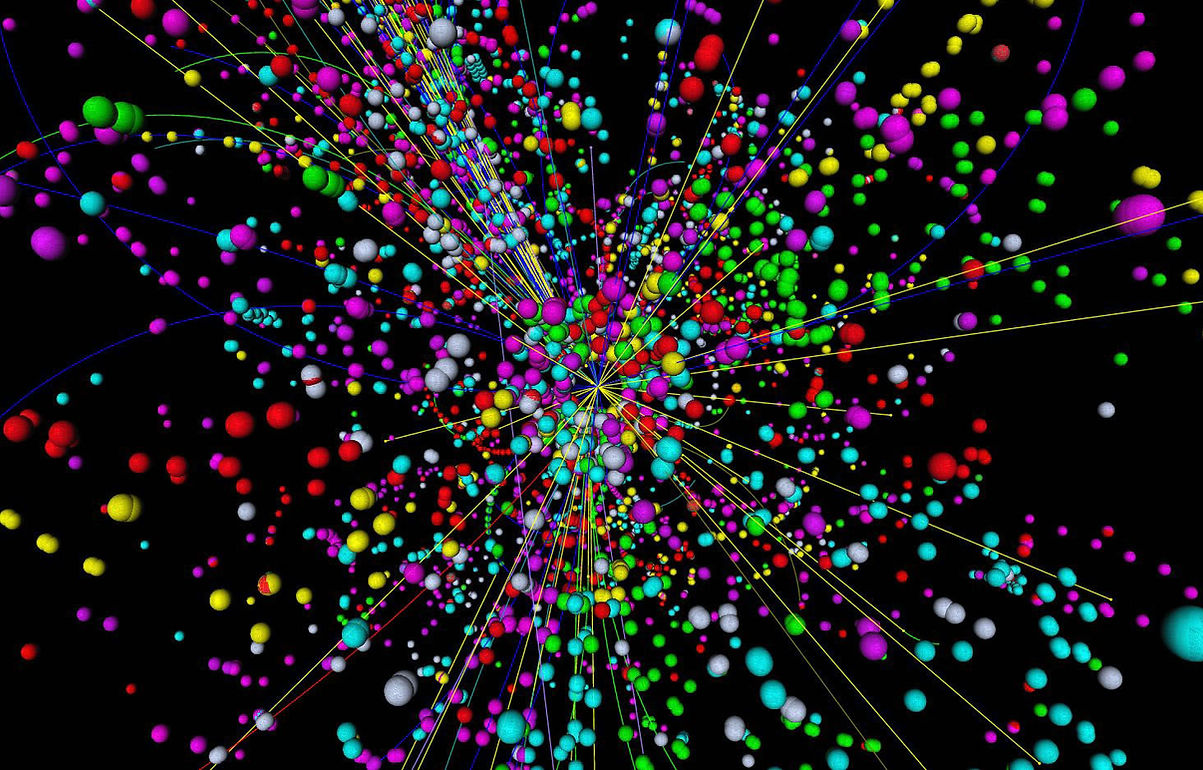
​
Although often overlooked, particle physics is one of the most important studies in science. It forms that base of the entire Standard Model. Without it, theories can be vague or wrong. Particles and quanta dominate our world. From day to day interactions (transfers of energy) to advanced computer science, our limit to our understanding of our world is only defined by our knowledge of these nanoscopic public servants. The most important of these: bosons.​
Bose-Einstein Statistics
Bose-Einstein Statistics is a phenomenon which describes how boson can and will behave at low temperatures. It works like this: When helium drops to 2.17o K, heat ceases its flow (shown right). At this temperature, some of the liquid becomes completely thickless, a zero viscosity that can skim over a surface in a density of less than an atom. This is known as a superfluid and is caused by all of the energy (bosons) leaving the helium atoms, and the helium then becomes void of energy. Along with this, the helium's electrons take on superconductivity
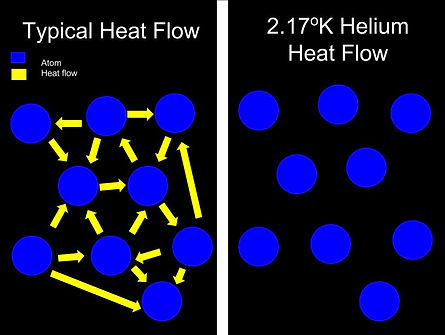
Bosons
Bosons are the force carriers in quantum physics. While fermions often have 1/2 spins, Bosons have full integer spins and are not subject to Pauli Exclusion (see below). Due to Bose-Einstein statistics (see below), groups of bosons must always stay symmetrical, a principle which ensures that force, light, and heat, travel equally in all directions. Bosons also can condense into a charge of 0 electric resistance.

in the absence of
This phenomenon can also happen with other elements, such as rubidium.
Types of Bosons
Gluons: Gluons mediate the strong force between quarks in hadrons and in nucleus between protons and neutrons. Gluons are suggested to be tri-colored , carrying a red, blue, and green charge and a color and anticolor for each of these. The bosons Gluons also interact, through the strong force, with each other this gives them mass by virtue of interaction. Gluons actually come from quarks. In order to mediate the strong force and avoid separation by entropy, quarks absorb and emit these bosons.
Photons: A photon is classified by light. These bosons only carry quantum light or electromagnetic radiation. This means that the photon is responsible for all the light on the color spectrum including X-rays and gamma radiation. Photons travel at almost exact celeritas and have no mass. All particle annihilation become photons with mass-energy. These then produce two leptons. Like gluons, photons are exchanged particles, but while gluons mediate quarks and baryons, photons mediate the electromagnetic force, carrying the signals through the air and diodes. They are responsible for keeping electrons orbiting their nucleases in atoms as well as particle spin and charge. It is the massless (at rest) photon that also gives the force infinite range. However, that does not mean photons don't have mass when stationary. Einstein's famous equation m=E/c^2 (more commonly E=mc^2) deems that all energy has some mass in the form of bosons and other related forces such as entropy, photons must have some weight. This value, however, varies extremely, based upon the amount of radiation and its frequency. The maximum mass of a traveling photon is 10−18 eV/c2. Photons were first realized in 1905 when Einstein realized the actual significance of Planck's work with the nanoscopic energy packets, quanta, and suggested a quantum carrier (or boson).
Gravitons: Until September 2016, gravitons were just an open theory. That was when gravitational waves were discovered at the Lazer Interferometer Gravitation Wave Observatory (LIGO). Gravitons are, as the name suggests, exchange particles for the gravitational field (spacetime curvature),. In other words, they transmit spacetime curvature, signaling to particles within the filled range to orbit/fall. Gravitons travel almost at the speed of light and have an energy-mass of 6x1032 eV. With the existence of gravitons comes the theory existence of antigravitons, a phenomenon in which spacetime would bend upward, speeding up time. The particles are massless and do not noticeably interact with matter.
Intermediate Vector Bosons: IVB confirmed the electroweak theory by describing how W and Z bosons control both the electromagnetic and weak forces. The existence of IVB was originally predicted in the 1960's by Sheldon L. Glashow, Steven Weinberg, and Albus Salam, however, these bosons' existences were not proved until 1984 by CERN physicist, Carlo Rubbia and engineer, Simon van der Meer who both won the Nobel prize for their discoveries. Both IVB only half-life 10-25 seconds.
W: with a positive charge and a huge mass of 80.4 GeV, the W boson is a key boson in the flavor change of quarks, sending positive (w+) or negative (w-) electromagnetic waves in the quark's direction. Over 1800 GeV of these bosons have been created in the Fermion National Accelerator Laboratory and the CERN Large Electron-Positron Collider.
Z: Only found in neutral form, the Z boson does not effect quarks flavors, but atom's interactions and decays. Weighing 109 eV, the Z boson ensures that during a particle's decay (weak force), energy is generated in photons are released ensuring both a weak and electromagnetic force, the electroweak force.
Fermions
Quarks
Quarks are the most common elementary particles in the universe. All with a spin of 1/2, quarks are responsible for the magnetic properties of elements and are found in all matter. There are six types, or 'flavors', of quarks and their associated antiparticles in order of decay. Note that the masses of these quarks, as well as the half-lives, are only a near estimate based on a series of unrelated experiments, as quarks cannot be confined.
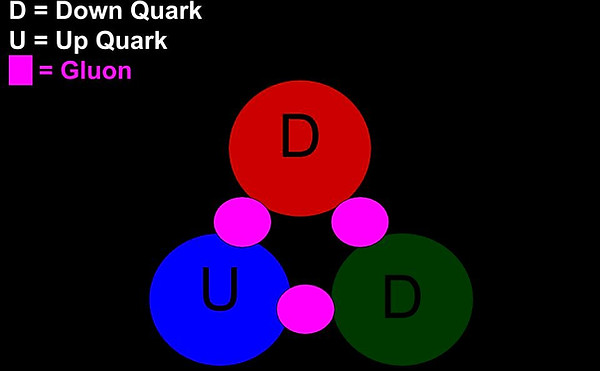
The Color Force
Quarks are very interesting in nature in that they not only have charge, but also color. Colors were introduced when a discrepancy was realized between the placing of quarks in hadrons and the Pauli Exclusion Principle. Among other things, the principle states that all particles of half-integer spin, such as quarks and electrons, cannot have the same quantum numbers, or essentially states of force arising from the solution of the Schrodinger Equation for when solved for hydrogen. In order to satisfy the Pauli Exclusion Principle and quark theory, quark color was hypothesized. Red, green, and blue were used to symbolize the three spatial quantum states of charge, orbital motion, and magnetic motion. The three colors, when combined, conveniently make white, so baryons like protons and neutrons would be 'colorless' or 'color neutral'. as shown right.
Leptons
While Quarks make up the more common, easily noticeable universe, Leptons consist of the smaller, shorter-lived particles. In fact, they are only formed through the annihilation of a quark-antiquark pair (meson), however, no Lepton will participate in any more than a weak interaction. An odd thing about these particles is size by mass doesn't seem to matter. A lepton could weigh as much as 20 kg and still be no larger than an electron. Unlike hadrons, leptons are always noted in their relative to the electron (their antiparticles positive). All leptons have a -1 charge of their form (for example, a muon has a -1 mV (muon volt)) and a 1/2 integer spin.
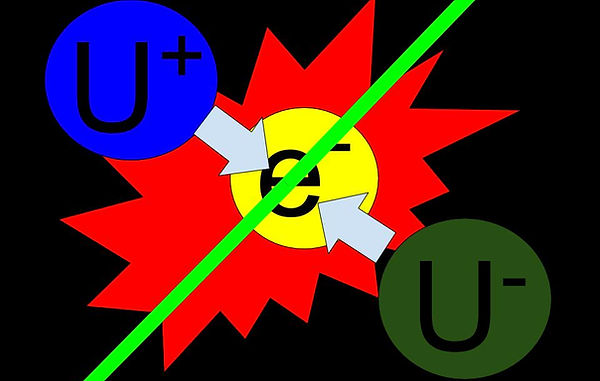
Hadrons
Made up of quarks and bound by the strong force, all complex particles (such as atoms) are made up of hadrons. All hadrons have a net spin and must be colorless. This includes baryons and pentaquarks, but technically not mesons, as they have no net spin and are thereby bosons. However, being made up of quarks the standard model considers them hadrons.
Baryons
Made up of three quarks, Baryons are likely the most common and useful particles in the universe
Mesons
Although they are made up of quarks, Mesons have zero integral spins and do not obey Pauli Exclusion making them bosons. However, the particles do not carry force and are not emitted or manipulated by fermions or energy. Thus, most physicists consider them hadrons. Generally, the particles are only used in the decays of baryons in order to fulfill conservation of baryon number without breaking conservation of energy. For example, a Delta (Δ+) baryon has 1232 MeV/c^2 of energy but decays into a Proton (P+) which has 939.57 MeV/c^2, a 292.43 MeV difference. Thus, in order to conserve energy, the baryon would have to release vast amounts of energy in the form of heat during decay, or also decay into a pion (π0) meson which conveniently has is 135 MeV/c^2, halving the energy lost through radiation. Mesons are made up of a quark-antiquark pair (such as up down) and have a baryon number of B=0. Although in a baryon, this would violate the strict laws requiring all hadrons to be colorless, the antiquark neutralizes its counterpart's color. For example, if one quark were green the antiquark would be antigreen by nature of interacting with the quark.
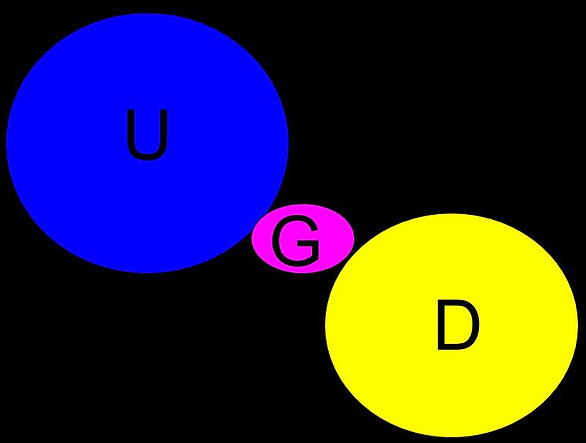