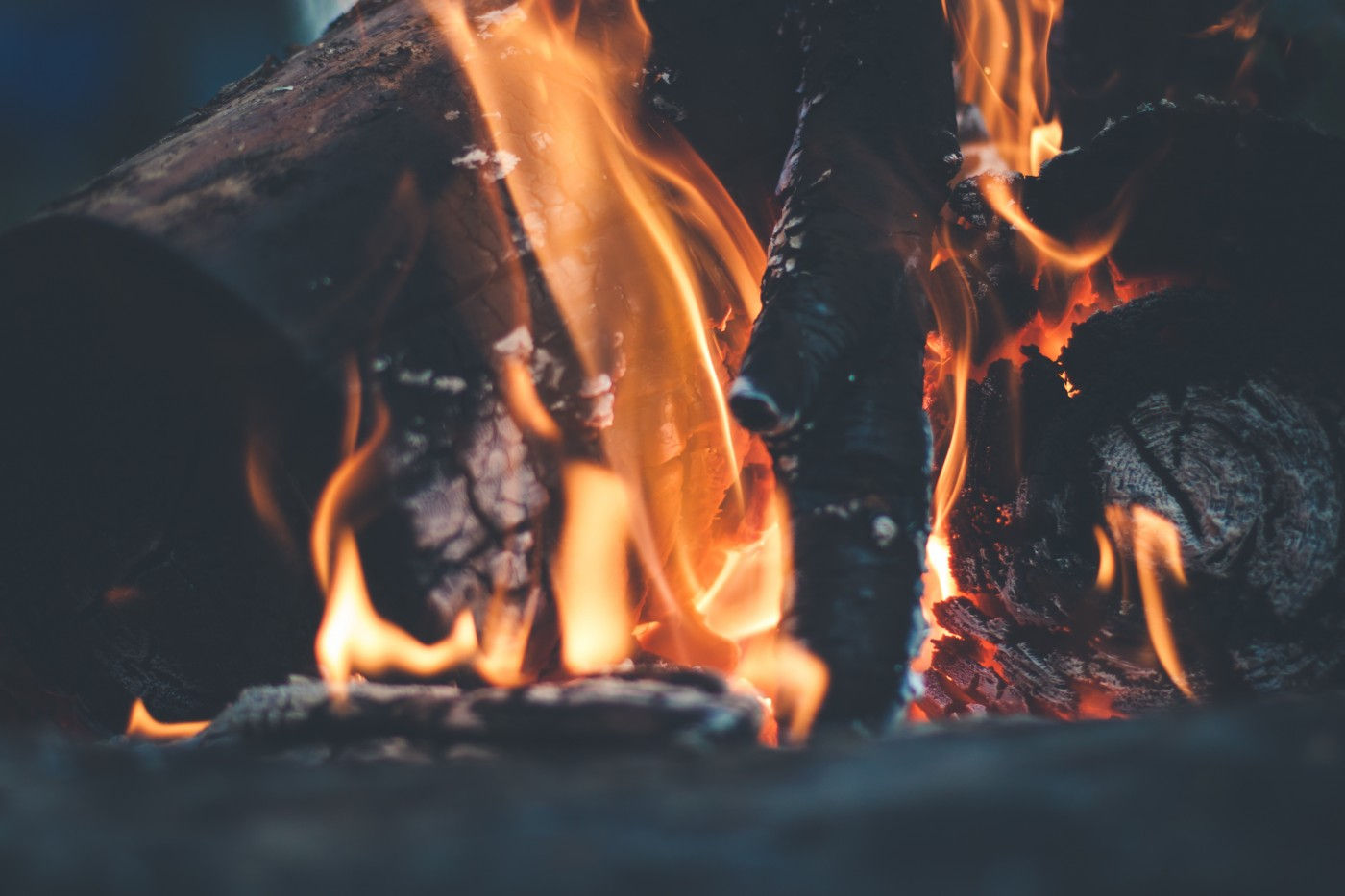
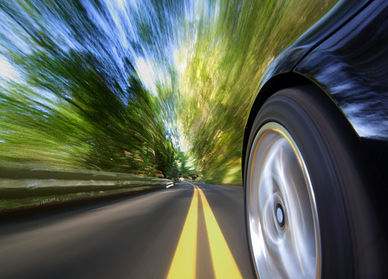
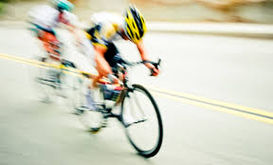
From athletics to transportation, to construction to central heating, kinematics governs our world. Although many overlook the subject as 'elementary physics', the study is absolutely necessary for human advancement. Kinematics is the study of how things move. How energy changes hands and even how particles interact. It is responsible for everything from entropy to sunburns but is crucial to our daily lives.
Kinematics
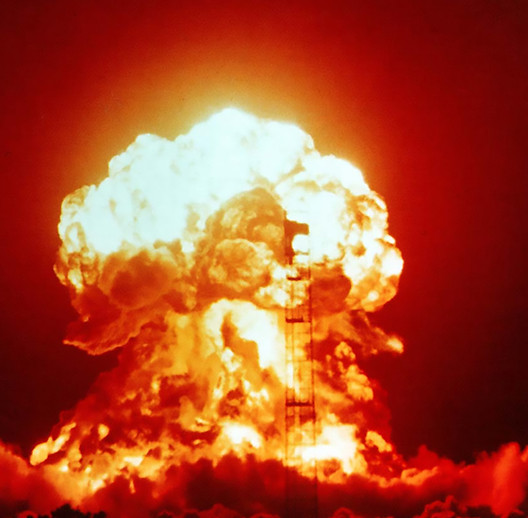
Fundamental Forces
There are four forces that govern the universe causing apples to fall, wind to move, stars to burn, and particles to decay:
Gravitational Force: As the weakest of the fundamental forces, but also the most common force. The Gravitational Force is transmitted by the graviton. Gravity is also the force governing acceleration. As yet discovered (if antigravity doesn't exist), g-force is always attractive and is a constant force, one of the first forces independent of the super force (see below). Though gravitons and gravitational waves never end and flow freely through all matter, a property only matched by the Higgs field, spacetime curvature itself, can be redesigned with dark energy and also eventually flattens out.
Strong Force: Why atomic nucleuses don't fall apart or fusion being the most powerful energy generator (almost 500 times more radiative than fission). This is the work of the strong force, by far the most powerful force the universe has to offer. The strong force binds particles with its exchange particle, the gluon. In fact, it would take a minimum of 1.112 MeV/A, to split the weakest atom, H-2. Though the gluon is the basic exchange particle, the pion, a meson, is actually the particle that travels between the strong interaction subjects (including repelling protons in a nucleus) to govern them, as gluons do not typically move. Due to π's mass, this force is typically slower to transmit than, say the electromagnetic force and also has the second lowest range working only approx. 1 fm away from its exchange particle.
Weak Force: Weak interaction has the smallest range of all the forces (however is not the weakest- there's a difference), only work within a width of 0.1% of that of a proton. However, this force is crucial to the success of nuclear fusion and flavor switch between quarks as well as particle decay. This force is necessary for the build up and creation of all the elements heavier than iron. The weak force is also essential to the success of the electroweak theory and was the last of the four independent force. The weak force is exchanged by intermediate vector bosons which release the force into hadrons. All particles participate in the weak interaction.
The Electroweak Force
In their normal interactions, the electromagnetic and weak forces both seem very different. The weak force has a range of less than an atomic nucleus while the electromagnetic stretches over the entire universe. The electromagnetic causes constructive motion while the weak force is often fighting that. However, in 1983 with the discovery of W and Z bosons came the phenomenon of a unified weak and electromagnetic force - the electroweak force. Before the universe started cooling, there was one super force (see below). As the universe began cooling the grand unified bosons began splitting. As it cooled, the Higgs field started breaking the universal symmetry causing W and Z bosons and photons began evolving into separate shapes thus causing separate weights and soon a split force.
​
The Grand Unified Theory (GUT)
Before even the electroweak force, the GUT was there. This grand force is the combination of the electroweak and strong forces. As the universe lost symmetry, most evidence of this theory was erased. There are a few problems with this theory. The electromagnetic force deals with leptons and charges while to be subject to the strong force, particles must have color, a phenomenon only possessed by
quarks. To solve this you'd need a boson that can convert leptons into quarks and back again. However, this breaks the conservation of the baryon number . To fix this you would need a boson that subtracts the lepton number from the baryon number, while still conserving the baryons' energy. To do this you'd need a boson that weighs at least 1015 MeV which would be more like the size of a skin flake than a nuclear particle. This boson is called boson X.
During the mid-1970s, physicists Sheldon Glashow and Howard Georgi proposed that boson X would be more a super particle than a boson. the GUT's force would generate a minimum of 1014 GeV. Using ordinary thermal energy concepts, this would require a temperature of over 1027 K- almost hotter than all of the generated energy is the galaxy in a hundred years! For this reason, no tests have been made to use the GUT and no boson X has been found.
The Supergrand Unification Theory (SUT)
Scene: 10-43 seconds after the big bang and a called the pre-Planck time. The universe is still undergoing inflation. No hadrons have formed and only the elementary particles. The universe hasn't even begun to cool. Space is still orange, but you wouldn't be able to tell, for the extremely jumble of fermions blocks all light, and bosons are not able to travel from one end of the microverse to the other as it is expanding faster than celeritas.
At this time in our universe's history, All four forces that govern our world are combined. Relativity and quantum physics did not form until transparent light and radiation could begin to start (100,000 years later). SUT has also been called the theory of everything- in which the universe is expanding so quickly, the forces can't lose symmetry. Not much is known from this time as the CMB could not form for another 300,000 years but further advances in particle accelerators could change that in the near future.
Electromagnetic Force: In 1865, James C Maxwell wrote 4 laws of electromagnetism
-
Gauss’ Law: Equal charges repel, opposites attract
-
Gauss’ Law of Magnetism: For every positive, there is a negative
-
Faraday’s Law: Electric fields wrap around a charge, the faster moving the charge, the faster the field rotates
-
Ampere’s Law: The more powerful the electric charge the bigger its magnetic field
The electromagnetic force is by far, the most affordable, efficient means of generating and storing energy, and also keep electrons from flying off into space, therefore, keeping things out of the hands of the weak forces. Before 1905 when Albert Einstein proved that electricity and magnetism were two different aspects of the same for forces in special relativity, it was believed that this force had a finite range and was subject to the governing of the strong force. Electromagnetism is an exchange force, using photons to transmit its signal at almost exactly the speed of light.
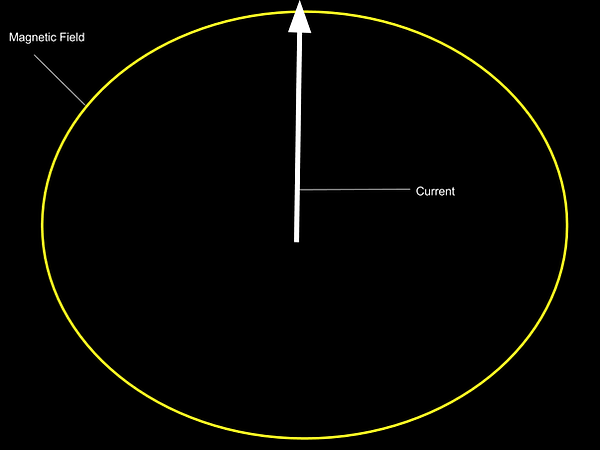
Mass-Energy
m=E/c2 says that, if you add up all the energies: K+T+T+P, and divide it by 8.98755179 × 1016 (c^2), you get the total extra mass of the moving train vs the unmoving train. Now granted, that's typically about 10-9% of the non-moving mass, but it still counts. After all, protons and neutrons would hardly weigh anything if it wasn't for the amounts of energy that make them up. Negative energy: A hydrogen atom weighs less than the sum of its parts (1 proton & 1 electron). Why is this? Unlike kinetic energy (as of 2016) potential energy can be negative. The farther away a proton is away from an electron, the more potential energy it has. The closer the fermions get, the less potential energy they have. For a hydrogen atom, the electron and proton are so close, their no additional attraction between them and the potential energy becomes negative. Now granted, the kinetic energy of the electron
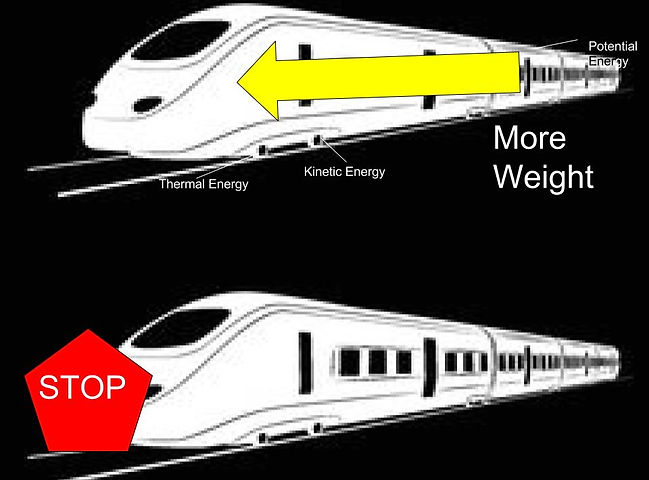
around the proton does make up for some of the energy debt, but not enough.
E=mc2 Albert Einstein's original equation for the relation of mass to energy was actually m=E/c2. This equation says that if I had two toy trains, identical atom for atom and one was moving, but the other had stopped, the moving one would weigh more. Einstein realized that energy must enact force upon objects to move it. However, in order to have force, one must have both mass and acceleration as described in the equation F=ma. Einstein realized that his mentor, physicist Max Planck was not only correct to use the math trick assuming energy is distributed throughout a substance in little packets of energy called quanta, he had inadvertently predicted the force carriers we call bosons today. Further evidence of Energy-Mass, mesons. Mesons are technically bosons. They are manipulated by the gauge bosons with non-infinite ranges to distribute the energy and complete a task. For example, since the strong force is too small to act over an entire nucleus, it emits pions which then carry out its bidding.
Mesons, just like baryons, are made up quarks. If baryons make up the visible world and are made up of quarks then mesons must have mass too. And how would a gauge boson emit a meson if it itself does not have mass? The principle of energy-mass was revolutionary to particle physics, enabling even more precise measuring tactics for future discovery.